Biosensors measure biological or chemical reactions by generating signals proportional to the concentration of an analyte in the reaction. Nowadays, they are a crucial aspect of biomedical diagnosis, as well as in other areas of point-of-care monitoring of treatments and progression of disease.
At Zimmer and Peacock, our applications of biosensors include point of care medical and veterinary diagnostics, as well as wearable biosensors. We have also reached the industries of food, agriculture and aquaculture with our biosensing technology. We know that it is not enough to produce just an electrode, we specialise also in functionalizing the electrodes to turn them into sensors; we specialise in adding enzymes, ionophores, antibodies, antigens, RNA, DNA etc to the surface of electrodes to give them specificity.
The first biosensor was developed by Leland Clark Jr in 1956 for oxygen detection, he became known as the ‘father of biosensors’. It wasn’t until 1975 that the first commercial biosensor was developed by Yellow Spring Instruments. Ever since the development of the handheld blood biosensor by i-STAT sensor in 1992 there has been remarkable progress within the biosensor field. The biosensor field has become a multidisciplinary area of research, combining the principles of basic sciences with the fundamentals of nanotechnology, as well as electronics and applicatory medicine.
The biosensor itself is typically made up of the following components. First is the analyte, this is the substance that is being detected. The bioreceptor is the molecule that recognises this analyte. Examples of biosensors inside the body include enzymes, cells and antibodies. The process of signal generation upon interaction with the bioreceptor with the analyte is called bio-recognition. Next, the transducer is the element converting the forms of energy through a process called signalisation, in this case it is used to convert the biorecognition event into a measurable signal. The electronics element processes this transduced signal and prepares it for quantifying on the display. Finally, the display consists of a user interpretation system similar to that of a mobile phone screen. This part consists of hardware and software that will display the generated results in a user-friendly way. The display may show a numeric value, an image or data in a table/ graphical form depending on the requirements.
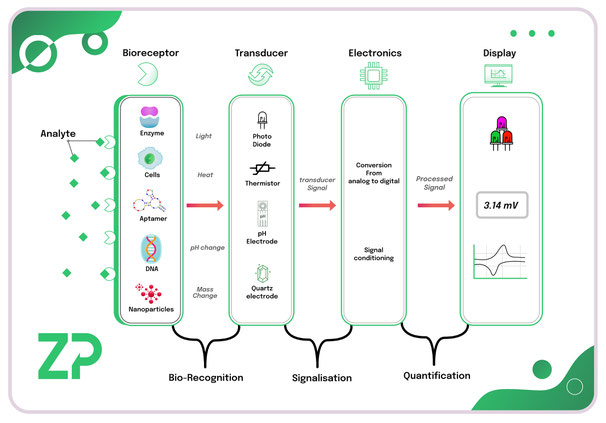
At ZP we feel that every biosensor should possess certain static and dynamic attributes. We understand that the performance of the biosensor will depend on the optimisation of these properties.
First of all, a crucial biosensor feature is stability, meaning in this case, the susceptibility to disturbances in the biosensing system. If present, any disturbance is likely to cause an obscurity in the output signal of the biosensor. Some examples of what may impact the stability include temperature sensitivity of the electronics, affinity of the biosensor and its degradation over time.
Arguably the most important feature of a biosensor is selectivity, the ability of a bioreceptor to detect the specific analyte in a sample containing other contaminants. The best example of selectivity is seen inside the body with the interaction of an antigen and an antibody. Antibodies tend to act as bioreceptors and are immobilised on the surface of the transducer. A solution (usually a buffer that contains salts) containing the antigen is then exposed to the transducer where antibodies only interact with the antigens. In the process of constructing a biosensor, the main consideration when choosing a bioreceptor should be selectivity.
Reproducibility is the ability of a biosensor to generate identical responses for duplicated experimental set-ups. The reproducibility is characterised by the precision and accuracy of the transducer and electronics in a biosensor. Precision is the ability of the sensor to provide the same results every time a sample is measured and accuracy indicates the sensor's capacity to provide a mean value close to the true value when a sample is measured more than once. Reproducible signals provide high reliability and robustness to the inference made on the response of a biosensor.
Another important feature is the sensitivity of the biosensor itself. The minimum value of analyte detected by the biosensor defines its sensitivity. This is a crucial characteristic of a biosensor since it could be required to detect analyte concentrations as low as ng/m.
The attribute of linearity shows the accuracy of the measured response to a straight line, in this case when using different concentrations of analyte. This is mathematically represented as y=mc, where y is the output signal, m is the sensitivity and c is the analyte concentration. The linearity of a biosensor is associated with the resolution of the biosensor and the range of concentrations of the analyte under test. The resolution is the smallest change in the analyte concentration that is required to bring about a change in the response of the biosensor. A good resolution is required across many different applications of biosensors, since most require measurement of concentration of analyte, not just initial detection.
Many biosensors have been created with the intention of improving quality of life. This can include their use in the areas of medicine, food safety, environmental monitoring and detection of disease. At ZP, our biosensors have also reached the industries of agriculture and aquaculture. Our focussed areas include point of care medical and veterinary diagnostics, as well as wearable biosensors. In the past, samples of blood, plasma, serum, urine etc from a patient would be collected and sent to a centralised lab for testing; the issue with this is that the doctor doesn’t get real-time feedback in order to make informed medical decisions. ZP is part of the movement to take clinical assays and move them into to doctor’s office and the patient’s homes, we do this by reducing cumbersome assays and diagnostics down to point-of-care-devices (POC) using electrochemical sensors, lab-on-a-chip and microfluidics. ZP also has a number of pre-existing sensors suitable for animal health applications; our team is able to partner with companies and academics interested in developing additional diagnostic tests and assays for the animal health and wellbeing market. The future of medical, wellbeing and sports performance is wearable sensors. At ZP we are ahead of the game with a wearable biosensor platform available to our customers and clients. Instead of focusing on standard parameters such as heart rate and steps, we are taking a different approach and focusing on glucose, lactate and hydration monitoring.
In terms of biosensor use in areas such as the food industry, these tend to be disposable and cost effective, as they are used on a singular occasion. However, in environmental monitoring processes, the biosensor will be required to work for a longer period of time, perhaps a few hours or days. At Zimmer and Peacock we understand these differences and are able to create biosensors with these specific requirements in mind.
Over the last few decades, biosensors have become attractive in both academia and in industry. The concept of combining biological recognition events with a transducing device brings together researchers from all areas of science and engineering. It has also allowed for new possibilities to open up in scientific research with attention drawn to development of technologies across multiple different industries including healthcare. Research in biosensors is not confined to a niche, as proven by the rapid increase in biosensors available on the market recently. Biosensors have a very important place in society and are no longer limited to a singular industry. Today are improving quality of life in a wide range of different areas such as pharmacology, food safety and homeland defence and security. At ZP our biosensors have even reached specific areas of agriculture such as fertilizer management for farmers.
Although the biosensor industry has been growing for the last few decades, very few biosensing devices have achieved global commercial success at retail level. Some possible limitations of producing commercial biosensors include identification of target market for a specific analyte, testing performance of a biosensor after storage or for long term use and the stability, cost and ease of component manufacture. There are also hazards and ethical issues associated with the use of certain biosensors. Increasing popularity of biosensing devices is meaning that these barriers are quickly being broken, with more and more research and education. With investments being put forward towards worldwide research for healthcare applications, industry is being brought closer to academia to provide commercially viable products. The alliance between researchers from many areas of development will inevitably result in huge advancements in biosensors. At ZP, our team is made up of researchers and engineers from all sorts of different backgrounds and we understand the impact that collaboration can have on something that started as a simple idea.
Development of good biosensing devices will require interaction across many different disciplines. At ZP we have a team of experts across multiple areas of science and engineering, allowing us to produce well-researched, top quality biosensors. ZP is able to work alongside clients to produce biosensors to specifically fit their needs.